Professor Chiara Ciccarelli
The Ciccarelli Group’s research focuses on the study of quantum spin-effects in magnetically ordered materials, from ferromagnets to anti-ferromagnets to chiral magnets.
Cold storage
Mind-bending speed. Extraordinary accuracy. And… no data storage to speak of. Quantum computing holds immense potential, but while quantum memory can store vast amounts of data, that storage is ‘non-persistent’ – it can only hang on to it for around 100 milliseconds. Oh, and did we mention it will only work below -273°C?
The year is 1900, and visitors to the Paris Exposition are charmed by a wonderful machine: the magnetic wire recorder. Its inventor, Danish engineer Valdemar Poulsen, uses his ‘telegraphone’ to record the Emperor Franz Josef saying: “Erfindung hat mich sehr interessiert, und ich danke sehr für die Vorführung derselben [I was very interested in the invention, and I thank you very much for demonstrating it].” This simple sentence heralds one of our age’s most revolutionary inventions: the magnetic storage system which made modern computers possible.
“And we still haven’t found anything else as cheap and stable as magnets for storing information for the longest period of time possible,” says Professor Chiara Ciccarelli of the Department of Physics. “There are, of course, other technologies that allow you to save information. But magnets can store it for decades without changing their magnetic state and therefore without losing the information.”

That’s why, more than 120 years later, Ciccarelli and her team at the Cavendish Laboratory have just been awarded a €2.1m (£1.75m) Consolidator Grant from the European Research Council which aims to push this extraordinary characteristic of magnets to its very limit. Her PICaSSO (Picosecond superconductivity-driven spin-torques) project will investigate one of the biggest conundrums facing the next great tech leap forward: why can’t quantum computers have a memory?
Of course, Ciccarelli explains, quantum computers are capable of incredible speeds and extraordinary accuracy. But while they can store vast amounts of data, they can only hold on to it for around 100 milliseconds. Then, it disappears. That’s because quantum computers are built with superconductors. These materials can perfectly conduct electricity without any energy loss – but only when they are cooled below -273°C, right above absolute zero. Therefore, quantum computers can only operate in these very cold temperatures. And as yet, nobody has worked out an efficient way to give quantum computers a magnetic memory that doesn’t involve heat.
“Just the act of a computer writing and reading magnetic information by controlling the direction of a magnet requires electrical currents,” says Ciccarelli. “These currents, in turn, produce heat. The computer can’t be connected to a magnetic memory storage device at room temperature, such as a server, because the act of connection – using a cable – would also transfer heat, rendering the quantum computer unusable. Right now, that’s why quantum computers are very small. To do anything meaningful with them, they need to be scaled up. For this to be possible, they need a memory.”
And there’s another problem: superconductors just don’t like magnets. In fact, they hate them so much that when they are brought into proximity, they will generate their own equal and opposite magnetic fields to push the magnet away – a phenomenon known as the Meissner effect. This disrupts the pairs of electrons – known as Cooper pairs – which carry electrical current in the superconductor. “Magnetism and superconductivity have long been regarded as two very incompatible states of matter: if you try to magnetise, you destroy superconductivity,” says Ciccarelli.
But, she points out, there is a lot more going on than scientists initially thought. Recent work has shown that in some cases, you don’t only rip apart Cooper pairs by introducing magnets. In fact, you can also create Cooper pairs that have aligned spins. The electrons continue to be superconducting and to be paired – and that means we can have magnetisation inside the superconductor.
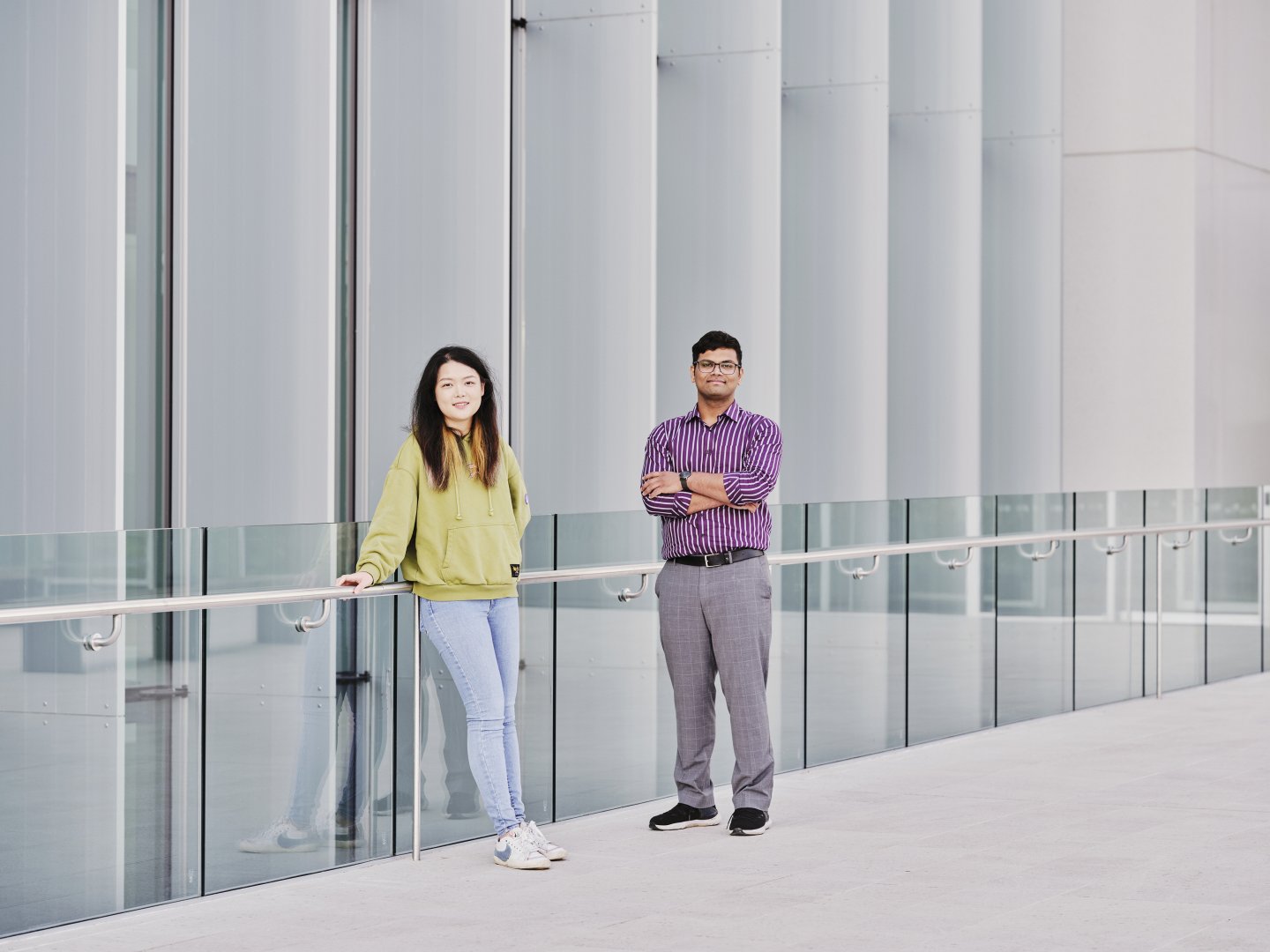
Zhuonan Wang and Ajinkya Pujal
Ciccarelli Group projects include: spin-orbitronics; superconducting spintronics; ultra-fast spintronics; and coupling between magnons and microwave phonons.
So, synergy between magnetism and superconductivity is actually possible. That’s what the PICaSSO project will examine: how well magnets and superconductors can ‘talk’ to each other, at incredibly fast timescales.
“If you put a magnet and a superconductor in contact and bring them together, their mutual energy is affected,” says Ciccarelli. “My idea is something new: to use this proximity to study the forces on the magnets which are generated directly at the interface with the superconductor, and therefore find new ways to read and write magnetic memories at low temperatures by using superconductors as active elements.”
How did she come up with the idea? It was a long, slightly messy process, she says. “For me, creativity begins with accessing a huge amount of information, and letting it sit throughout a period of absolute confusion. And at some point, you emerge from this confusion and in a moment everything seems to be in place again. It’s a pattern I’ve noticed throughout my life.”
Ciccarelli’s fascination with magnets came with her own moment of clarity after she completed her MSc at the University of Rome Tor Vergata and was looking for a PhD subject. Her professor gave her a paper on spintronics – spin electronics, the study of magnets. “I liked the word,” she says. “And the physics behind magnets is really nice: there is a lot of quantum mechanics involved. I started looking for a group, which I found in Cambridge, and I have been here ever since.
I wanted to contribute something to the scientific community which very few people can do. I realised that there was a gap in the research. Nobody has yet studied the proximity structures between magnets and superconductors at fast timescales.
“It was a shock when I arrived, though, as my background was in purely theoretical physics, and I had to learn to use my hands in the lab for the first time. You have to make magnetic devices that are pretty small.” How small? “About a millionth of a metre. Or, if you like, a thousand times smaller than a millimetre. You have to practise a lot with the tweezers. It’s a bit like cooking: you follow a recipe but also develop intuition on what needs to be done to make something better.”
Her interest in superconductors came later, thanks to a collaboration with Professor Mark Blamire and Professor Jason Robinson at the Department of Materials Science and Metallurgy. “This is where we found out about how Cooper pairs could align. And I saw the potential for this to merge with my other interest: terahertz spintronics – essentially, studying magnets at much faster timescales. We want to know how fast we can read and write the magnetic state, to have faster memories.” (We are, incidentally, talking very fast timescales here: right now, it takes around 10-9 seconds to write a magnetic unit of memory: Ciccarelli wants it to take 10-12 seconds. “I wanted to contribute something to the scientific community which only I can do – or very few people can do,” she says. “I realised that there was a gap in the research. Nobody has yet studied the proximity structures between magnets and superconductors at fast time scales.”
The PICaSSO project officially started in June and will last for five years. “We can suppress superconductivity very quickly by sending a terahertz pulse – a very rapid electric field pulse. When this happens, the superconductor becomes a normal metal very rapidly. It then returns to its superconducting state, and this process takes tens of picoseconds. I would love to see the magnet near it flip or change its magnetic direction as we do this, because this tells us that we can do what we had in mind – use superconductors as active elements to control the magnet.”
The potential impact is as extraordinary as the imperceptible quantum forces that surround us: computers of incredible power, with the capacity to store infinite amounts of information. “Quantum mechanics brings a level of free will, if you like: it allows for infinite possibilities living together in the same state, and you pick one of these possibilities via interaction,” says Ciccarelli.
This still-mysterious realm is something she thinks about a lot. “It’s interesting to consider that our brains, too, are a superposition of possibilities, and we get to choose. A normal computer is deterministic: once you have the inputs, an algorithm gives you the answers. But we are not deterministic: perhaps we are closer to a quantum computer.” She laughs. “This is too philosophical. But who knows what impacts quantum laws might have on us in the future? Right now, we only perceive the macroscopic physics around us. But that has nothing to do with true physics, governed by quantum laws.”
To learn more about supporting these projects, please contact Amy Bleasdale.